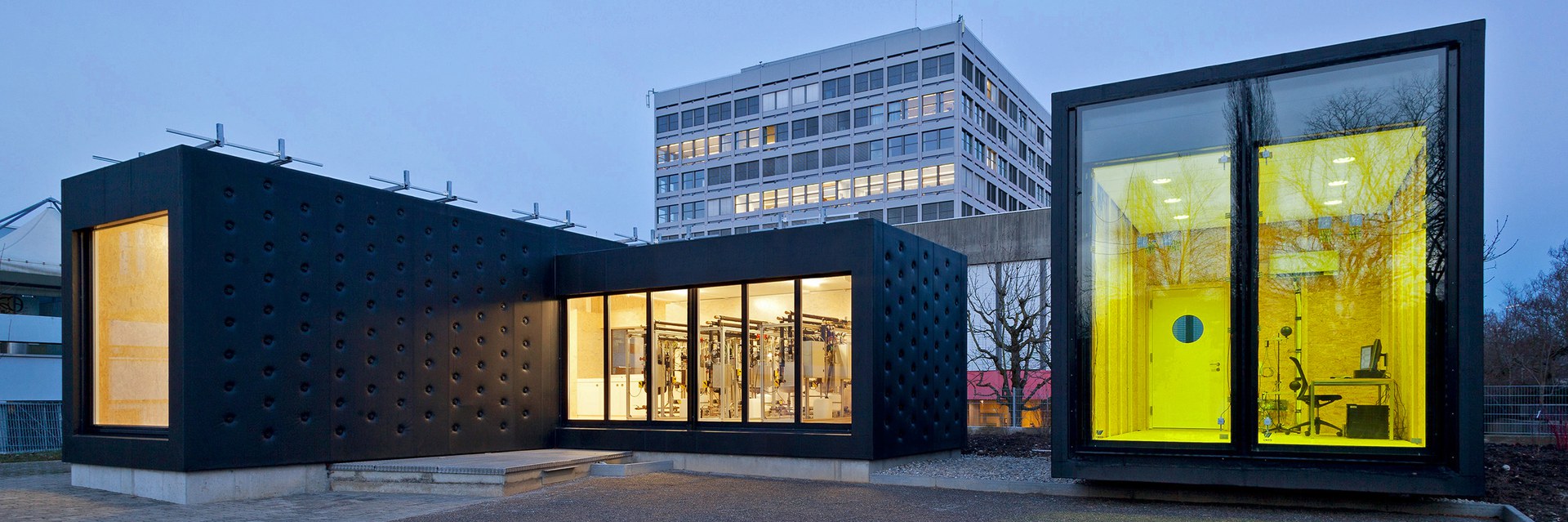
Thermal Hydraulic Methods
for Energy Systems in Buildings and other applications
In energy systems, phenomena of fluid dynamics, thermodynamics, and heat and mass transfer usually occur simultaneously and in mutual dependence, which must be considered in the design and operation of such systems. Only then is it possible to minimize use of materials, and to optimize energy efficiency and costs while at the same time achieving operational reliability.
These goals can be achieved by a thermohydraulic approach, which integrates thermodynamics, fluid mechanics and heat transfer in an interdisciplinary way. The thermohydraulic approach has many advantages over the disciplinary approach one normally finds in textbooks.
Interdependencies in the development of thermohydraulic models:
Planning sustainable energy systems and developing their components is not only a great challenge that engineers, product developers and planners should joyfully accept, but also a vital duty. To decarbonize the building energy sector, the operation of these systems must be based on renewable energies. But this alone is not enough. To achieve sustainability, additional conditions must be met:
- The optimum of energy efficiency and costs
- No oversizing of systems and components. Low use of materials, recyclable design
- Good part-load performance
- Trouble-free operation, durable, easy to maintain
- Optimum function because of careful planning rather than a result of subsequent adjustments.
Achieving these goals requires a model-based approach to product development and planning. This is the leitmotif of this course!
Product development and Plant design are interdisciplinary tasks, because knowledge and skills from different fields of engineering and economy is required.
To find optimal solutions, stakeholders must be involved, e.g., end users, operators, investors, workers, salespersons, installers. Therefore, a purely disciplinary approach, even if interdisciplinary, is not sufficient. Sustainable solutions can only be achieved through a transdisciplinary approach that accounts for the different goals and perspectives of all stakeholders.
How does Transdisciplinarity appear in the course?
- Teaching content is always embedded in the technical and societal context.
- The context is made tangible through numerous stories and use cases from practice. These include not only success stories, but also failures, from which, as is well known, one learns the most.
Topics covered in the course
- Each topic will be motivated by one or several practical use cases for which we will derive appropriate models.
- No specific previous knowledge is required. Mathematical methods will be introduced when they are needed.
- Many models are based on current research results and are therefore not yet found in textbooks.
- With the knowledge gained in the lecture you will be well equipped to design sustainable energy systems.
If you have questions, don’t hesitate to ask:
Prof. Dr. Ralph Eismann | FHNW
In the following sections, some topics are briefly outlined and illustrated:
- Pipe friction is an important phenomenon in pipe networks.
- For pipe network simulations to converge, pressure loss correlations must be continuous functions of the Reynolds number!
- We will discuss suitable correlations and use them to solve real-world tasks.
- We will discuss how to generate models for variable speed pumps based on data sheets.
- We will also generalize the inlet pressure requirements so that only temperature and altitude above sea level are required.
- As with all topics we cover, the pump model is represented by a computer program. As you will see, this will prove very handy in plant modeling.
In the classical sense, thermohydraulics means the simultaneous solution of the conservation equations for mass, momentum and energy. We will discuss different methods of pipe network analysis and apply them in practical examples by using free open-source tools provided by the lecturer.
The figure shows a prime example for thermal hydraulics: the flow- temperature-, and pressure distribution in a solar thermal collector array.
- Correctly functioning pressure maintenance is a basic prerequisite for the fault-free, efficient operation of a hydronic system.
- Unfortunately, established dimensioning rules are subject to considerable uncertainties that can lead to system failures.
- You will learn a new, model-based method that considers uncertainties of pressure measurement as well as the effect of vessel temperature.
- A practical free and open-source tool is provided that can be used for dimensioning and maintenance.
Based on the NTU-formalism we will derive a new model that is able to generalize data-sheets of fan coils!
We extend this to a model for circuit-coupled heat recovery systems and use a practical tool to simulate full and part load operation under different boundary conditions.
- Free gases cause operational problems and must therefore be removed from the circuit.
- To achieve this, the gases must be transported to the air separator by flow forces.
- We will learn methods to calculate the so-called self-venting velocity. This will be very helpful in dimensioning and routing of pipe networks!
We will dimension the evaporator of a heat pump, using methods for calculating the two-phase
- Void fraction
- Pressure loss
- Heat transfer
Kontakt
-
Prof. Dr. Ralph Eismann
- Telefonnummer
- +41 61 228 53 61 (Direkt)
- cmFscGguZWlzbWFubkBmaG53LmNo
- Fachhochschule Nordwestschweiz FHNW
Hochschule für Architektur, Bau und Geomatik
Institut Nachhaltigkeit und Energie am Bau
Hofackerstrasse 30
4132 Muttenz - Raum 11.W.25